COCONUT
The coconut palm, Cocos nucifera L. grows best under conditions of high humidity, at temperatures of 27–30˚C and on moderately to well-aerated soils. Coconut is the most extensively grown and used palm in the world. Coconut is mainly an oil crop, particularly rich (48 %) in lauric acid. Virgin coconut oil, expelled under low heat from fresh coconut meat to preserve its natural vitamins and enzymes is now becoming popular in the pharmaceutical industry and is gaining a significant international market.
Coconut as a bio-fuel is also a newly emerging product which would reinstate coconut palm as a valuable oil crop in the not too distant future. In addition, the coconut industry offers a wide range of coconut products such as desiccated coconut, coconut cream, coconut milk powder, defatted coconut, coconut fibre and fibre products, shell charcoal and activated carbon, coconut vinegar and coconut arrack (liquor) etc. for the local and the international markets. Tender coconut as a natural beverage is presently gaining popularity and currently there is also a growing international market for fresh coconut water. Coconut oil is also the principal raw material used in the manufacture of soap, glycerine and margarine. The lauric acid from coconut oil is used to manufacture detergents, cosmetics and pharmaceuticals. Locally the trunk is made into furniture, handicrafts and building materials, the fibre from the nuts for mattresses, doormats and ropes. Because of the multiplicity of uses of the coconut palm, it is termed ‘one of Nature’s greatest gifts to man’ and also as ‘The Tree of Life’.
Cocos nucifera L. is a member of the monocotyledon family Arecaceae (Palmaceae) in the subfamily Cocoideae that includes 27 genera and 600 species and is the only species of the genus Cocos. Coconut possesses a diploid genome with 32 chromosomes (2n = 2x = 32). There are conflicting theories regarding the origin and domestication of coconut.
Molecular markers
Application of molecular genetics in coconut breeding, particularly molecular markers began in the early 1990s and their application in coconut have been diverse, ranging from assessing genetic diversity to creating genetic linkage maps. Initially the studies were aimed at assessment of coconut genetic diversity and genetic relatedness at the DNA level using universal marker techniques such as RAPD, RFLP, AFLP and ISTR. Later on the need for coconut specific markers was felt and accordingly in 1999 two sets of microsatellite markers were isolated by two groups of scientists independently using the cultivars Sri Lanka Tall and Tagnanan Tall. Microsatellites as co-dominant markers have been particularly useful in analyzing highly heterozygous coconut for genetic diversity and genetic relatedness estimates, germplasm characterization and development of collections, hybridity testing, detecting somaclonal variation in coconut tissue cultures, and for construction of genetic linkage maps. A microsatellite kit comprising 14 primers and an associated software for data analysis has also been developed standardizing the techniques across laboratories for comparable results, efficient detection of diversity and identification of varieties. Among these markers, SSRs have been the most widely and extensively used in analyzing coconut.
Genetic Diversity Analysis
A high level of genetic diversity in coconut has been observed using microsatellites in a collection of 130 coconut individuals sampled across the entire geographic range in the world. An recent study, found a reduced number of alleles in dwarf coconut group compared to tall coconut group, comparable with a reduction in the amount of genetic diversity in dwarfs. From the results, they concluded that there had been a low but variable rate of gene migration between South Pacific populations with possible founder effects and subsequent human selection.
In a study, constructed a phenetic tree diagram showing the genetic relationships among 51 tall coconut varieties and 49 dwarf coconut varieties across the world. Instantly this phenetic tree divided all tall coconuts into two main groups, the first group comprising all the tall varieties from Southeast Asia, the Pacific and the west coast of Panama and all dwarfs in a sub-cluster within the tall cluster. The second group consisted of talls from South Asia, East Africa and West Africa. Interestingly, none of the dwarf coconuts grouped with the second main tall group.
Studied 17 different coconut varieties from different geographical regions and identified groupings of African coconut with Indian Ocean coconuts and Panama Tall coconut from the west coast of Panama with Pacific and Southeast Asian coconuts. Lebrun et al. (1998) reported the use of RFLPs in coconut from various geographical regions. Results from all these studies finally led to the conclusion that Cocos nucifera is divided into two large genetic groups, the Southeast Asia and Pacific group and the Indo-Atlantic group.
The genetic relationships among 33 coconut germplasm accessions were analyzed using RAPD markers. Forty-five random primers produced a total of 399 polymorphic markers. There was less genetic similarity (based on Jaccard’s coefficient) among South Pacific and South East Asian accessions. The clustering pattern obtained was in agreement with the earlier reports based on RFLP, SSRs and AFLPs.
Genetic differences between genotypes tolerant to lethal yellowing disease and the susceptible ones were evaluated using twelve microsatellite markers. The work aimed to use identified materials as reference to select suitable parents for gene mapping studies. A total of 58 alleles were detected at the 12 microsatellite loci. The number of alleles varied from 3 to 7, with an average of 4.83 alleles. The Fst index revealed that 59.70% of the total allele variability explained differences between the three accessions. Genotypes of West African Tall, susceptible to the lethal yellowing disease, were less genetically clustered to the genotypes of the two tolerant accessions. This differentiation was based on specific alleles and frequency variation of shared allele in the three accessions. This molecular typology was useful as reference for large molecular screening of coconut genetic resources and the identification of suitable parents for the development of mapping populations for tagging the lethal yellowing resistance genes.
Cloning One of the genes identified in early somatic embryogenesis is SOMATIC EMBRYOGENESIS RECEPTOR-like KINASE (SERK). The SERK ortholog was referred to as CnSERK. Predicted sequence analysis showed that CnSERK encodes a SERK protein. Analysis showed that it could be detected in embryogenic tissues before embryo development. In contrast it was not detected or at lower levels in non-embryogenic tissues, thus suggesting that CnSERK expression is associated with induction of somatic embryogenesis and that it could be a potential marker of cells competent to form somatic embryos in coconut tissues cultured in vitro.
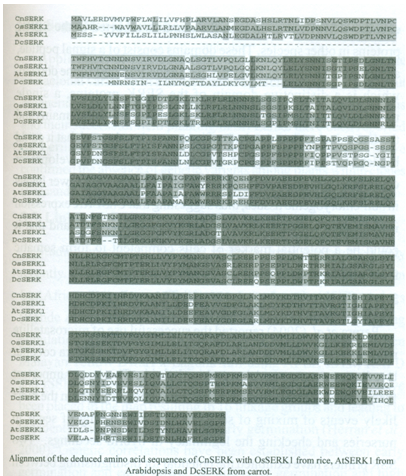 |
Hybridity Testing and Variety Identification
The successful use of microsatellite markers to uniquely identify seed parents in coconut breeding programme and the resulting hybrids. This is very important for confirming the identity of varieties and their hybrids in the most likely events of mixing of seed-nut lots, mislabeling of seeds in nurseries and checking the legitimacy of hybrid seedlings. Two SSR primers have exhibited the potential for distinguishing between coconut varieties Sri Lanka Tall, Sri Lanka Green Dwarf and Sri Lanka Yellow Dwarf used as parents in Sri Lanka. This has led to the unique capability of conformity and hybridity testing of two commercially grown coconut hybrids, Sri Lanka Green Dwarf x Sri Lanka Tall and Sri Lanka Yellow Dwarf x Sri Lanka Tall.
Linkage Mapping and QTL Identification
Studies on coconut genome mapping have commenced only recently. The first genome map for coconut was developed for an East African Tall x Laguna Tall F1 population based on ISTR markers. This work was extended with a mapping population developed in the Philippines from a cross between Malayan Yellow Dwarf x Laguna Tall using AFLP, ISTR, RAPD and ISSR markers. Three hundred and eighty two markers have been placed in the map resulting in 16 linkage groups and leading to the identification of six QTLs for early germination. Further, QTL for other traits such as leaf production, girth and height have also been identified for the same mapping population. In addition to this, another mapping population in Ivory Coast derived from Cameroon Red Dwarfs and Rennell Island Tall has been used to map 280 markers on 16 linkage groups resulting in several QTLs related to nut number, number of bunches and traits related to fruit components.
Cuticular waxes were extracted from the leaves of a coconut mapping population generated by the controlled cross of an East African Tall and a Rennell Island Tall genotype for the construction of molecular linkage maps. The wax composition was analyzed by capillary gas chromatography/mass spectrometry, and for eight of the wax compounds, their absolute and relative amounts were determined. The variation detected for parents and progeny with respect to the wax components allowed quantitative trait locus (QTL) analyses for their biosynthetic pathways. A total of 46 QTLs could be mapped onto the coconut linkage map which was extended by amplified fragment length polymorphism and single sequence repeat markers into a high density map with more than 1,000 mapped DNA markers.
In vitro Culture
Coconut tissue culture has a long history dating back to the 1970s. Initial attempts were on reversion of floral meristem into vegetative growth; but later on, most efforts were concentrated on somatic embryogenesis. During the past decade, the in vitro plant regeneration protocol has been improved, and a limited number of clonal plantlets obtained mainly from zygotic tissues in a few laboratories. However, success is very limited due to numerous constraints. The regeneration efficiency is far from adequate though a small number of vegetatively propagated coconut palms have been established in the field. Due to very poor response of coconut tissues to in vitro conditions it is classified as one of the most recalcitrant species to regenerate in vitro.
Tall coconut palm, an allogamous and variable species, is highly heterozygous which is a major constraint in conventional breeding. Availability of homozygous lines would improve the efficiency of generating new cultivars through breeding programs. Gametic embryogenesis allows single-step development of completely homozygous lines from heterozygous parents. Although the production of haploids by microspore embryogenesis has been reported for many crop species, haploid plant production from anthers, or isolated microspores of woody species has had a low success rate. Even though the studies on coconut anther culture have been initiated since the 1970s, complete plant regeneration has not been reported. For the first time that there is a feasibility of developing homozygous lines in coconut via anther culture. Growth regulators are critical for controlling the androgenic response. The type and concentration of auxin has an effect on the frequency of callus yield, the proportion of albino plants regenerated from calli and the frequencies of dihaploids among green plants recovered.
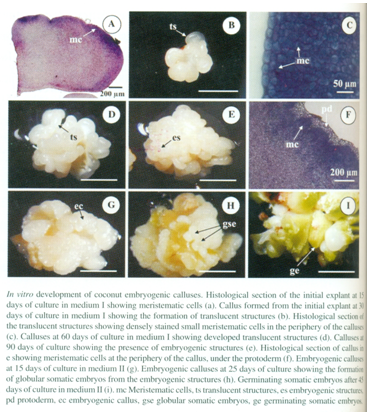 |
Simple sequence repeat (SSR) marker analysis was used to identify the homozygous diploid individuals among dihaploid plantlets obtained via anther culture. Using a diagnostic SSR marker (CNZ43), all the diploid plantlets tested were identified as being derived from microspores (i.e. were homozygous) and were thus candidates for use in coconut breeding programs.
Plant regeneration via anther culture of coconut (Cocos nucifera L.). (a) Embryos (em) derived from anther (an). Note the tiny connector (arrow head) between the embryo and the anther wall. (b) Mature embryo with germination point (gp). (c) Germinating embryo with a shoot (s) emerging by splitting of haustorium (h). (d) Calli (ca) bearing anther. (e) An anther bearing both callus (ca) and embryos (em). Note the embryo is opaque white whereas the callus has a translucent appearance . (f) Somatic embryos (se) developed from anther derived callus. (g) A complete plantlet derived from anther.
Consistent callogenesis was obtained by culturing unfertilised ovaries in CRI 72 medium containing 100 µM 2,4-dichlorophenoxyacetic acid (2,4-D) and 0.1% activated charcoal. Embryogenic calli were subcultured onto somatic embryogenesis induction medium containing 66 µM 2,4-D. Stunted growth was observed in the somatic embryos after subculture onto CRI 72 medium containing abscisic acid (ABA). Maturation of somatic embryos could be achieved in Y3 medium without growth regulators. Conversion of somatic embryos to plantlets was induced by adding gibberellic acid (GA3) to conversion medium containing 5 µM 6-benzyladenine (BA) while 2-isopentyl adenine (2iP) increased the frequency of plant regeneration. A total of 83 plantlets were produced from 32 cultured ovaries.
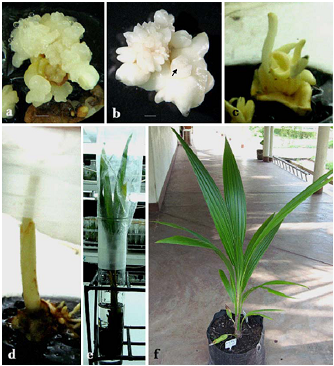 |
Plant regeneration via ovary culture of coconut (Cocos nucifera L.). a Ovary-derived callus produced in callogenesis induction medium. b Somatic embryos obtained after subculturing the calli onto somatic embryo maturation medium devoid of growth regulators. Note the converted somatic embryo indicated by an arrow. c Early stage of shoot development of a converted somatic embryo. d Shoot and root development of the plantlet in conversion medium containing GA3 that was maintained in the dark. e Well developed complete plantlet in liquid conversion medium containing GA3. f Ovary-derived coconut plant transferred to the soil.
Crop improvement programmes in coconut led to development of hybrids, collection, conservation and cataloguing of germplasm. Collection of germplasm using different tissue culture techniques, DNA fingerprinting and MAS are the developments in biotechnology of coconut. Biotechnology can be used to improve coconut in several ways. By improving our knowledge of coconut diversity, molecular methods could help breeders effectively choose varieties to be used in a breeding programme to maximise heterosis and the chances of incorporating useful genes. It could also be used to identify markers for quantitative trait loci (QTLs), i.e. individual genes that contribute significantly to the diversity of a quantitative trait. Finally, if a large number of inexpensive markers is available, association mapping can be performed in natural or breeding populations without the need for a precise knowledge of the pedigrees.
Source: Dr.V.Ponnuswami, PhD, PDF (Taiwan), Former Dean & Professor (Horticulture), Horticultural College & Research Institute, Tamil Nadu Agricultural University, Coimbatore
|